StarVIBE
StarVIBE provided a comparable image contrast to BW-CT (Figure 1). Its radial k-space sampling proved robust to patient and internal organ motion. However, stellate artefacts from the air in the bowel were frequently observed (Figure 2). While these artefacts were visually distracting, they did not significantly impede bone visualisation.
The main drawback of StarVIBE was its long acquisition time (TA: 06:00 per slab). Additionally, its image resolution was inferior to that of BW-CT. The lengthy acquisition time, driven by the number of radial views needed for adequate SNR and resolution, made StarVIBE impractical for whole-body imaging despite its motion robustness.
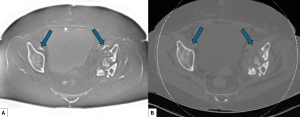
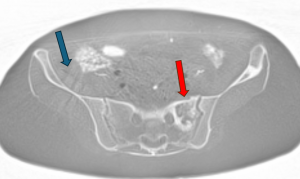
Breath-hold (BH) VIBE
We discarded the Cartesian-sampled BH VIBE approach due to poor SNR and resolution. This resulted from the low flip angle and the short breath-hold duration (maximum 00:20) necessary for patient comfort (Figure 3A). Motion artefacts shifted from stellate to linear along the phase-encoding direction, which breath-holding largely mitigated. We noted that breath-holding BH VIBE could be potentially useful for visualising lesions in the ribs and sternum.
BH DL VIBE
Deep learning reconstruction dramatically improved image quality in the breath-hold VIBE sequence. BH DL VIBE significantly reduced noise and enhanced resolution compared to the standard BH VIBE (Figure 3B).
While BH DL VIBE improved image quality, its spatial resolution remained inferior to that of BW-CT. Applying a sharp filter marginally improved image sharpness (Figure 4), but the images still fell short of BW-CT quality.
Increasing the acquisition matrix to match BW-CT spatial resolution resulted in acquisition times exceeding breath-hold tolerance and introduced additional noise, leading to a loss of cortical detail (Figure 5). We observed that detail loss from noise could be mitigated by increasing signal (e.g., by increasing the number of excitations or flip angles). Still, the resulting longer acquisition times would be impractical for breath-hold imaging.
Clinically, higher image resolution was preferred for a closer resemblance to BW-CT, so the breath-hold constraint was relaxed.
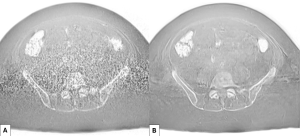
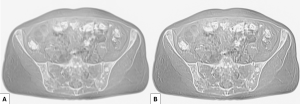
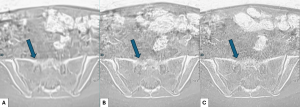
Free-breathing DL VIBE
Using a 384 matrix, reducing acceleration factors, increasing excitations (number of signal averages, NSA) from 1 to 2, and disabling slice and phase partial Fourier, we improved SNR and BW-CT-equivalent resolution with free-breathing DL VIBE. This resulted in a TA of 02:04 per station. While some motion artefacts reappeared, the TA was significantly better than StarVIBE, and image resolution was improved (Figure 6).
We explored reducing excitations from 2 to 1 to reduce acquisition time further. However, this proved unfavourable in patients with higher body mass index (BMI), as illustrated in Figures 7 and 8.
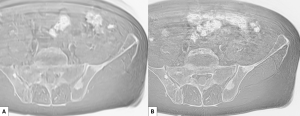
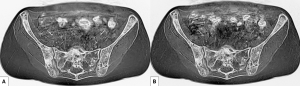
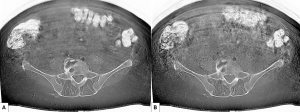
Limitations
MRI bone detail was compared to abdominopelvic CT using body-kernel reconstruction. Our MRI method has not yet achieved the superior sharpness of bone-kernel CT.
All tissues with low signal appear white on inverted images, similar to bone. Consequently, air and low-signal structures like tendons and ligaments can be difficult to differentiate from bone.
Cartesian gradient echo sequences also suffer from chemical shift artefacts, seen as "cartooning" or outlining at the bone-soft tissue interface. Performing an in-phase acquisition eliminates the majority of chemical shift artefacts. Increasing receive bandwidth can reduce these artefacts further but degrades SNR (Figure 9). While aesthetically undesirable, the residual chemical shift artefacts on in-phase imaging were not considered clinically significant as they did not obscure bone visualisation.
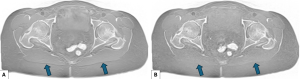
Whole-Body (WB-MRI) applications:
For whole-body bone imaging, we established two options: high-resolution imaging with longer TA for regions requiring detailed assessment and lower-resolution imaging within breath-hold duration for mobile anatomy like the chest and upper abdomen. These techniques can be combined across WB-MRI stations by ensuring consistent image resolution.
Implementing WB-MRI requires additional considerations. (1) Slab composition can introduce mismatches in continuous bone structures on multi-planar reformats (MPRs) (Figure 10A). (2) 3D distortion correction is necessary to minimise geometric shifts of bone and tissue at slab edges (Figure 10B). (3) Edge slices can exhibit zero signal from non-existent "adjacent" slices, necessitating extra slices and increased TA. (4) Optimising slab overlap is crucial for generating high-quality coronal and sagittal MPRs despite the 2mm slice thickness.
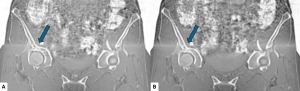
Clinical case 1:
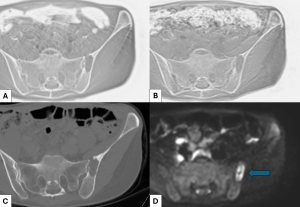
Clinical case 2:
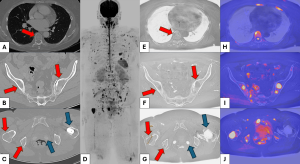
Key sequence parameters and technical notes:
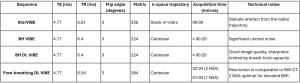